Serena Eley wins NSF CAREER Award to develop building blocks of next-gen spintronics, quantum computing
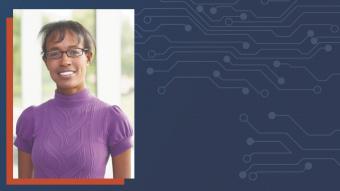
Serena Eley, assistant professor of physics at Colorado School of Mines, has received a National Science Foundation CAREER award for work that could help design a new architecture for next-generation spintronics and quantum computing devices.
A rapidly advancing research area, spintronics explores methods to exploit a property of electrons – called spin – to encode information faster and in a more energy efficient fashion than conventional computing electronics.
Eley will receive $640,887 over five years for her project, “Skyrmion-Vortex Interactions in Ferromagnet-Superconductor Heterostructures,” which proposes to develop promising platforms for both superconducting spintronics and topological quantum computing.
Here, Eley answers a few questions about her research and how a new instrument at Mines will allow researchers to not only infer the dynamics of vortices and skyrmions from electrical measurements, but also image them.
Q: What is your latest research focused on?
A: My research is generally focused on understanding the effects of disorder on the electronic and magnetic properties of quantum materials and devices. Specifically, we study vortex-defect interactions in superconductors, skyrmion-defect interactions in magnetic materials, and the effects of the material microstructure on energy loss in superconducting circuits.
Vortices are topological excitations that appear in many different systems, including superconductors, magnets, superfluids, liquid crystals and Bose-Einstein condensates. In superconductors, vortices are typically unwanted as their motion greatly limits the current carrying capacity in superconductors and induces microwave energy loss in superconducting quantum circuits. On the contrary, in certain chiral magnets and magnetic multilayers, vortex-like excitations (nanoscale whirlpools of magnetic moments) called skyrmions are beneficial for use as information carriers in next-generation low-energy spintronic devices. We seek to develop a microscopic understanding of the complex interplay between vortices, material disorder, and thermal energy to mitigate the deleterious effects of superconducting vortices and exploit skyrmions in spintronics.
In this NSF CAREER project, our goal is to design, fabricate, and study superconductor-ferromagnetic heterostructures that could host skyrmion-vortex pairs. Ferromagnet-superconductor heterostructures are promising platforms for devices in superconducting spintronics and topological quantum computing, two potentially transformative research areas. I proposed a couple different device schemes in which skyrmion-vortex pairs are likely to form, and these pairs are theorized to support Majorana modes – elusive states that are desirable for use in fault-tolerant quantum computation. We will employ magnetic force microscopy and electrical transport measurements to study the dynamics of magnetic textures that emerge in these devices under variable temperatures (down to 1.6 Kelvin) and magnetic fields (up to 12 Tesla), determining the conditions under which skyrmions and vortices co-exist and bind. We will further study the effects of thermal energy and current-induced forces, comparing results to semiclassical theories describing skyrmion-vortex pair dynamics.
Q: What do you find most exciting about your research?
A: One of the most exciting activities in our new effort will involve imaging nanoscale magnetic structures using magnetic force microscopy (MFM), an upcoming new capability at Mines that was enabled by a 2019 Major Research Instrumentation grant from the National Science Foundation. We typically infer the existence of vortices and skyrmions from fitting our magnetization and transport data to models. However, using the MFM, we will be able to image vortices and skyrmions. We will host the new instrument in my lab, and it will be broadly available to researchers internally and throughout the Front Range.
Q: What is the potential impact of this work?
A: The broader impacts of the proposed work include designing next-generation building blocks for spintronics and fault-tolerant quantum computing. The field of spintronics is a rapidly advancing and disruptive research area, producing novel device schemes that are superior to conventional computing electronics.
Conventional electronic elements use electron charge to store and transmit information. For example, the amount of charge accumulated on a capacitor may determine whether it contributes a “0” or a “1” binary digit. On the other hand, spintronic devices exploit another property of electrons, called spin (up, down, or canted), to encode information. These devices can in fact use spin currents to control logic operations faster and more energy efficiently than charge currents can accomplish in conventional semiconductor transistor-based logic.
Spintronics devices typically consist of ferromagnets as the source of the spin current. Incorporating elements that are superconductors, which have no electrical resistance, can enhance the overall device performance and offer new functionalities, such as remarkably low-dissipation (energy loss) spin transport. Such ferromagnetic-superconductor structures and quasiparticles hosted therein are also constituents of schemes for topological quantum computing, an architecture that is predicted to be robust to environmental noise that plagues other quantum device platforms.
Q: How does this research agenda inform your teaching?
A: I am incorporating more fundamentals of Solid State physics into my Analog Electronics lectures to provide students with a stronger foundation to approach specialized topics relevant to my research. The proposed work also includes opportunities for contributions from undergraduates through Senior Design and the Mines Undergraduate Research Fellowship program. Furthermore, we will continue to work with Peter Aaen in Electrical Engineering to design a new lab course for the Quantum Engineering Master’s Program that teaches low-temperature and microwave measurement techniques. It also includes providing training in electronics and robotics to K-12 students through partnerships with a local elementary school.